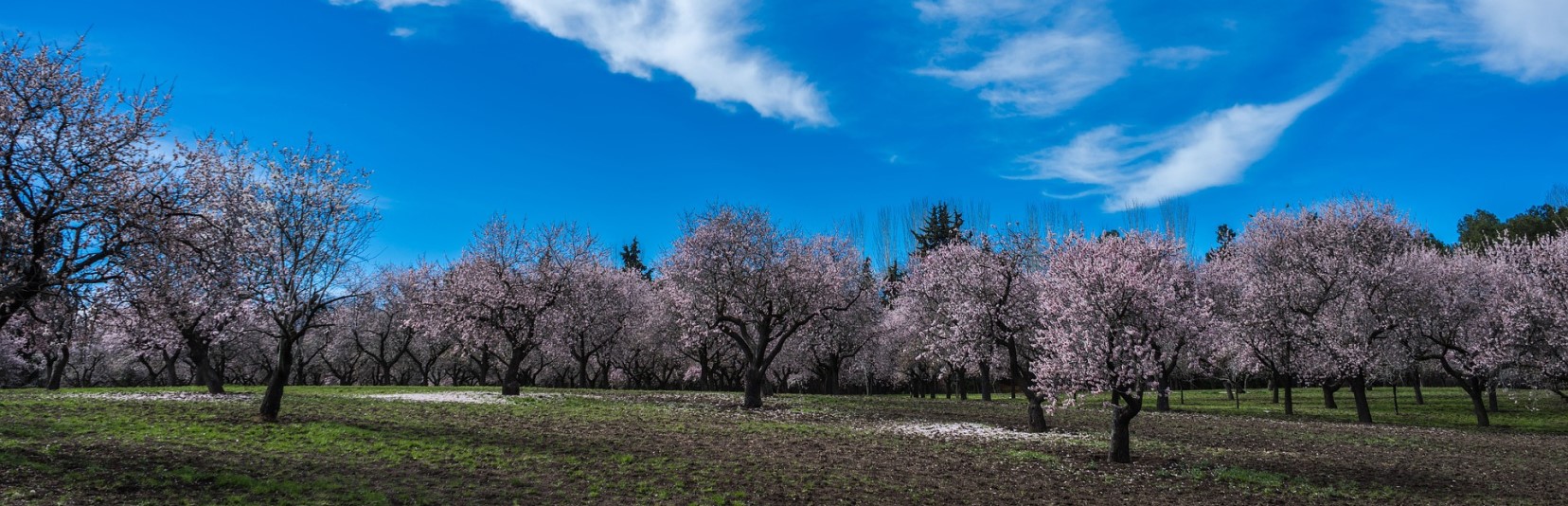
Almond Crop Biology & Growth Requirements
The almond tree thrives in the Mediterranean climate, adapting to a wide range of soil and weather conditions. While it tolerates high summer temperatures, intense winter cold, drought, and even poor soil, these factors will ultimately affect its yield. The better the environmental conditions, the higher the potential almond production.
Crop/Flower Biology
For almond growers, flowering time is critical. Early blooms risk frost damage, impacting yields. Because successful pollination in almonds requires overlapping bloom periods, understanding the precise timing of floral development events becomes even more crucial.
Flowering will only occur once dormancy has been broken. Endodormancy, primarily influenced by the balance of plant hormones and non-structural carbohydrate reserves, refers to the tree’s inability to initiate floral or vegetative bud break despite suitable temperatures. Endodormancy precedes ecodormancy, which takes place during late winter and spring and is triggered by temperatures that are not conducive to growth.
Unlike other Prunus species that solely rely on spurs, almond trees boast a unique ability to produce flower buds in two locations. The primary site is on short, specialized branches called spurs, where each spur can hold up to six individual flower buds. These spurs remain productive for an average of 3 to 5 years. Interestingly, unlike vegetative buds that focus on leafy growth, flower buds lack leaves entirely. They dedicate their resources to nurturing a single terminal flower, ready for pollination. Additionally, almond trees can also develop flower buds laterally on more vigorous shoots from the current year, offering further potential for flower production. The number of flower buds produced at a single node (point where a shoot or spur connects to the stem) can vary not only between different almond cultivars but also within the same cultivar itself.
(source: https://doi.org/10.1080/14620316.2002.11511525).
Unlike most plants where development progresses in a specific direction (base to tip, tip to base, or both), flower bud formation on almond tree spurs happens independently. This suggests that individual buds on a spur may not rely on each other for development and might be influenced by factors like local sugar availability from nearby leaves or the specific vascular connections between leaves and that particular spur.
(source: https://doi.org/10.1080/14620316.2002.11511525).
Almond flower structure varies among cultivars. Studies have shown that the position of the stigma (female reproductive organ) can be above, below, or level with the highest anthers (male reproductive organs). Additionally, the average number of stamens (pollen-bearing structures) can differ significantly, ranging from 17.3 in some selections (e.g., Tree No.2) to 39.8 in cultivars like ‘Gaura Almond.’ Interestingly, the length of both pistils (female) and stamens (male) can also vary considerably. Despite this variation in flower morphology, research suggests no clear link between flower structure and a tree’s ability to self-pollinate (self-compatibility).
Variety Selection: Important considerations
Important characteristics to consider in almond variety selection include time of bloom; pollen compatibility; time of maturity and ease of nut removal; yield; freedom from pests, diseases, and other disorders; and marketability (including kernel quality).
Bloom Time:
Bloom time is crucial in assessing a variety’s frost damage risk and pollination success. Early-blooming varieties may face more frost and poor pollination conditions. Proper overlap of bloom times is essential for effective cross-pollination. Almond varieties are categorized by bloom periods in Table 3. Varieties within the same or adjacent bloom groups (e.g., Nonpareil and Carmel) generally cross-pollinate well, except in incompatible cases (see Table 4). Closer bloom timing improves cross-pollination chances due to better flower receptivity overlap and bee activity. However, early- and late-blooming varieties (e.g., Ne Plus Ultra and Mission) may not overlap enough for effective pollination. Mid- and late-blooming varieties (e.g., Nonpareil and Mission) often have inadequate overlap. The relative bloom order is also significant, especially for the main variety and its pollenizers. For instance, Ne Plus Ultra, which blooms 6 days before Nonpareil, is a better pollenizer than Mission, which blooms 5-7 days later. Bloom times can vary annually and by location due to temperature fluctuations before and during bloom.
Table 3: Almond varieties grouped by approximate bloom periods.
Note: This table is based primarily on results of the Manteca Regional Variety Trial. The numbers in the column heads indicate the days before (–) or after (+) peak Nonpareil bloom. (Source: The Evaluation and Selection of Current Varieties; https://ucanr.edu/sites/kingscounty/files/18977.pdf).
Pollen Incompatibility: Almond varieties can be categorized into specific groups based on their pollen compatibility. Varieties within the same group are generally cross-incompatible, meaning they cannot pollinate each other. However, varieties from different groups are typically cross-compatible and can successfully pollinate each other. The exception to this rule is the Jeffries variety, which exhibits reciprocal incompatibility within certain groups. This means that Jeffries cannot pollinate or be pollinated by varieties within those specific groups. Tables 4 and 5 provide a detailed overview of these compatibility relationships. (Source: The Evaluation and Selection of Current Varieties; https://ucanr.edu/sites/kingscounty/files/18977.pdf).
Table 4: Pollen-incompatible groups of almond varieties.
* Jeffries, being a mutation of Nonpareil, should theoretically belong to the Nonpareil incompatibility group. However, field observations and controlled tests from 1984 and 1985 reveal that Jeffries exhibits unilateral incompatibility. While all varieties, including the parent Nonpareil, can fertilize Jeffries, Jeffries cannot fertilize Nonpareil, Carmel, Solano, Monterey, and other varieties in these incompatibility groups, nor Butte. Conversely, Jeffries can fertilize all varieties in the Mission, Ne Plus Ultra, and Thompson groups, as well as Fritz.
Table 5: Varieties tested for pollen incompatibility, for which no separate incompatibility group has been identified.
* If a variety from a known incompatible group is listed as compatible, then all other varieties within that group should also be compatible.
Time of Maturity and Ease of Nut Removal: Time of maturity and ease of harvest (see Tables 6 and 7) are crucial for variety selection. Understanding when varieties mature helps manage harvest labor and equipment efficiently, minimizing quality loss and exposure to pests and weather. Planting varieties with similar maturity times allows for well-timed preharvest irrigation and orchard preparation. Late-maturing varieties face higher risk from poor weather and potential damage. The sequence of maturity generally remains consistent year-to-year and by location. Ease of nut removal is also important to avoid tree damage and additional costs. Leftover nuts can attract pests and cause further damage to the tree. (Source: The Evaluation and Selection of Current Varieties; https://ucanr.edu/sites/kingscounty/files/18977.pdf).
Table 6: Almond varieties’ time of maturity (readiness for harvest).
*Indicates approximate number of days after Nonpareil maturity. †LeGrand may harvest better at an earlier date (or a double harvest may be helpful).
Table 7: Relative simplicity of nut removal for almond varieties.
Yield: While yield potential is critical, it varies based on the year, location, pollination, orchard management, and other factors. (Source: The Evaluation and Selection of Current Varieties; https://ucanr.edu/sites/kingscounty/files/18977.pdf).
Marketability: Different varieties are categorized for marketing purposes. Mixing varieties can reduce husking capacity and market value. Understanding marketing categories and consulting with handlers is essential to optimize crop pricing and value. (Source: The Evaluation and Selection of Current Varieties; https://ucanr.edu/sites/kingscounty/files/18977.pdf).
Phenological Stages
Phenological Stages:
The main phenological stages of an almond tree typically include (see Fig. 1):
- Dormancy: During winter, almond trees enter a dormant phase, shedding their leaves and conserving energy.
- Bud Swell: As temperatures begin to rise in early spring, buds on the almond tree start to swell in preparation for blooming.
- Bloom: Almond trees produce beautiful blossoms, attracting pollinators to facilitate fertilization.
- Fruit Set: Following successful pollination, the almond tree’s flowers transition into developing fruits, known as almonds.
- Fruit Development: Throughout the spring and summer months, the almond fruits mature and grow in size on the tree.
- Fruit Ripening: The final stage involves drying of the kernel and splitting of the hull, allowing for harvest.
- Harvest: Almonds are typically ready for harvest in late summer to early autumn, depending on the variety and location of the almond tree.
- Leaf Senescence: With the arrival of fall, almond trees undergo leaf senescence, preparing to enter another dormancy phase as winter approaches.
Fig. 1. Almond different phenological stages includes: 1. Dormancy; 2. Bud Swell; 3. Bloom; 4. Fruit Set; 5. Fruit Development; 6. Fruit Ripening; 7. Harvest; 8. Leaf Senescence.
Growth Requirements:
Dormancy has a major economic impact on almond maintenance and production. Insufficient chilling can decrease productivity across various almond cultivars. Typically, low production or uneven flowering follows unusually mild winters. Insufficient winter chilling or inadequate soil moisture during bud development often causes floral buds to shed. About 20 to 30% of floral buds may be lost after an exceptionally mild winter, with even higher losses in particularly sensitive cultivars like ‘Peerless’ and ‘Ne Plus Ultra’.
The detrimental effects of inadequate chilling on dormancy breaking in temperate fruits, including almonds, have been well-documented. For optimal vegetative growth and fruit-bearing, almond cultivars must meet their chilling requirements. Conversely, in areas with low chilling requirements, such as where early-flowering cultivars grow in cold winters, premature blooming can lead to significant yield losses due to frost.
Flowering timing in almonds is considered a polygenic trait, influenced by factors like the previous year’s yield, harvest date, environmental conditions (e.g., dry or wet winters), and chilling hours (CUs). Various models estimate chilling and heat hour requirements for almonds, with thresholds typically defined by hours below 7°C and above 10°C.
Data suggests that flowering timing is predominantly influenced by chilling requirements rather than heat requirements.
In regions with ample CUs, flowering tends to be more synchronized due to quicker fulfillment of chilling requirements. Some new almond cultivars with higher chilling needs pose a risk in very warm areas with inadequate chilling temperatures. Although late-flowering cultivars may have slightly higher heat requirements, chilling requirements primarily determine flowering time. Consequently, breeding efforts for late-flowering almonds should focus on crossing cultivars with high chilling requirements. Regional maps assessing the chilling and heat requirements of new areas could be valuable for determining the feasibility of growing new cultivars.
Climate:
Temperature and Almond Tree Health
Optimal Temperature for Growth:
- Temperate zone fruit trees, like almonds, thrive in a sweet spot between 25-30°C for photosynthesis. This process slows down significantly below 15°C or above 35°C.
Winter Chilling Needs:
- Almond trees require a period of winter cold to initiate proper growth, flowering, and fruit set. This need is quantified using “Chilling Hours” (CH), which represent the total time the tree spends below 7°C.
- Almonds have relatively low CH needs (between 100-400 hours depending on variety), similar to other Mediterranean fruits like figs and olives.
Cold Tolerance:
- Almond trees are among the most winter-hardy fruit trees, tolerating temperatures as low as -15°C during dormancy. However, their vulnerability increases during flowering and fruit development:
- Freshly formed fruits are most susceptible to frost damage, followed by open flowers and buds (damaged at temperatures slightly below 0°C).
- Early flowering varieties are particularly at risk of frost damage. Planting late-flowering cultivars like ‘Arquero’ is recommended in frost-prone areas (Arquero, 2013).
- Pink buds can tolerate temperatures down to -4 to -6°C, but open blooms are damaged at -2°C.
High Temperatures:
Excessive heat can also harm almond trees. As mentioned earlier, photosynthesis decreases significantly above 35°C, potentially leading to a summer dormancy period, especially during dry conditions.
Even higher temperatures (above 40°C) can cause dehydration, tissue death (necrosis), leaf drop, fruit damage, and even wood scorching.
Rainfall as a Challenge:
Almond production in Mediterranean climates is significantly hindered by erratic and insufficient rainfall. Historically, these regions have experienced prolonged summer droughts often extending into spring and autumn, exacerbated by frequent dry years. Climate change has further compounded these challenges by altering rainfall patterns. Previously predictable rainfall has become increasingly intense but shorter-lived, with extended dry spells. Consequently, the traditional irrigation practice of withholding water during the typically rainy early spring and summer, when almonds flower and set fruit, is no longer reliable. The heightened risk of water stress during these critical growth stages can compromise current and future yields. To address this, advanced technologies for monitoring soil moisture, plant responses, and weather conditions are essential to refine irrigation strategies during these vulnerable periods.
Almond Tree Adaptation:
While well-adapted to rainfed conditions, almond trees significantly increase their yield with irrigation. In areas receiving less than 500mm of annual rainfall and experiencing prolonged droughts, irrigation becomes crucial to maintain production levels.
Relative Humidity: A Balancing Act
Relative humidity (RH) plays a significant role in almond health. Both very high and very low RH can cause problems:
- High RH: When humidity is too high, it can lead to stomatal closure, hindering the plant’s ability to take in CO2 for growth and reproduction. Additionally, high humidity favors the spread of diseases, potentially limiting crop success.
- Low RH: While almonds don’t necessarily need high humidity, excessively dry conditions can also be detrimental.
Managing Humidity Through Cultivation:
Fortunately, we have some control over RH within the orchard through cultivation practices. Techniques like using foggers or overhead sprinklers can:
- Increase humidity: Misting by the use of foggers above the tree canopy can add moisture to the air, particularly beneficial in drier regions to prevent excessively low RH.
- Improve air circulation: Strategically placed sprinklers can create a cooling effect and enhance air movement within the orchard, indirectly helping to manage humidity levels.
Also, designing the plantation layout and implementing specific tree training and pruning methods can help:
- Decrease tree and canopy density: This allows for better air circulation within the orchard, helping to reduce humidity levels and improve ventilation.
Climate: Vapor Pressure Deficit (VPD) and its Impact on Almond Tree Performance
Vapor pressure deficit (VPD) is a crucial environmental factor significantly impacting almond tree performance. It represents the difference between the saturation vapor pressure (the amount of water vapour the air can hold at a specific temperature) and the actual vapor pressure (the amount of water vapor currently present in the air).
In recent decades, rising global temperatures have led to a significant increase in vapor pressure deficit (VPD). VPD has become a crucial factor in plant functioning across terrestrial biomes and is a major contributor to drought-induced plant mortality, independent of other climate change factors. Evidence indicates that under high VPD, stomatal conductance generally decreases while transpiration increases up to a certain VPD threshold. This results in a series of effects, including reduced photosynthesis and growth, and an increased risk of carbon starvation and hydraulic failure.
Here’s a breakdown of its influence:
Stomatal Conductance and Transpiration:
- Stomata: Tiny pores on the underside of leaves that regulate gas exchange (CO2 intake and water vapor and oxygen release) between the plant and the atmosphere.
- Impact of VPD: High VPD creates a strong “pull” on water molecules within the plant, initially prompting the stomata to open wider to facilitate water vapor release through transpiration. However, when a certain threshold is reached, the stomata start to close to minimize water loss through transpiration.
- Increased Transpiration: High VPD directly increases transpiration rates in almond trees. As the difference between the water vapor content of the air and the tree’s leaves increases, water is lost more rapidly through transpiration.
- Water Loss: Excessive transpiration can lead to water loss from the tree, which can negatively impact its health and productivity.
Water Stress and Plant Health:
- Increased Transpiration: As VPD rises, transpiration increases, leading to greater water loss from the plant.
- Water Stress: If the rate of water loss through transpiration exceeds the rate of water uptake from the roots, the plant experiences water stress.
- Impact on Almond Trees: Water stress in almond trees can negatively affect:
- Photosynthesis: CO2 uptake is reduced due to partially closed stomata to conserve water.
- Growth and Development: Growth slows down, potentially leading to smaller fruit size and reduced yield.
- Nutrient Uptake: Water stress can hinder the ability of roots to absorb nutrients effectively.
- Susceptibility to Disease: Water-stressed trees are more vulnerable to insect pests and diseases.
Optimal VPD Range for Almonds:
Almond trees are relatively drought-tolerant, but they still have an optimal VPD range for ideal growth and performance. This range can vary slightly depending on the specific growth stage, age and cultivar, but it generally falls between:
Day time:
- Lower Limit: Around 1 kPa (kilopascal)
- Upper Limit: Around 3 kPa
Nighttime:
- Lower Limit: Around 0 kPa (kilopascal)
- Upper Limit: Around 0.6 kPa
Strategies to Mitigate High VPD:
- Irrigation Management: Generally, during the day, when the stomata are open, the rate of water loss through transpiration can exceed the plant’s or tree’s ability to absorb water from the soil. As a result, plant or tree tissues tend to dehydrate throughout the day, losing size (a few tens to hundreds of microns) and creating a negative water potential gradient from the root (less negative) to the canopy (more negative). This negative gradient allows the plant or tree to continue absorbing water at night, even when the stomata are closed. Consequently, at night, the plant or tree tissues begin to rehydrate, regaining or increasing their size (a few tens to hundreds of microns) if water availability or nighttime VPD are not limiting factors. Top of Form
- Bottom of Form
So, proper irrigation practices are crucial for maintaining adequate soil moisture and reducing water stress during periods of high VPD. To optimize tree health during periods of high upcoming VPD (day or night), consider strategic late afternoon or early evening irrigation for your almond trees to maximize tissues rehydration during the night. This timing can also help them replenish water reserves before the increased evaporative stress hits.
- Canopy Management: Techniques like pruning to promote air circulation and shade the orchard floor can help create a more favorable microclimate around the trees.
- Selection of Drought-Tolerant Cultivars: Choosing almond varieties known for better drought tolerance can be beneficial in areas with high VPD conditions.
Monitoring VPD:
Monitoring VPD levels throughout the growing season allows growers to implement proactive measures to minimize water stress on their almond trees. Weather stations or dedicated sensors can be used for VPD measurement.
By understanding the relationship between VPD and almond tree performance, growers can optimize irrigation practices and orchard management techniques to ensure healthy trees and maximize yields.
Impact of High Day/Nighttime Vapor Pressure Deficit (VPD) on Almond Trees
High day/nighttime VPD, may induce tree stress, however, high night VPD may sometimes, have more significant negative impacts on almond tree performance. Here’s a breakdown of the specific concerns for high day/night VPD:
Reduced Photosynthesis:
- Stomatal Closure: During the day, high VPD can cause stomata to close prematurely, leading to reduced CO2 uptake, photosynthesis, and energy reserves. At night, high VPD can hinder tissue rehydration, causing plant/tree water stress the next day, especially if VPD remains high or increases.
- Limited Carbon Gain: Reduced CO2 uptake during the day directly translates to lower carbohydrate production, a crucial energy source for various plant functions, including growth, flowering, and fruit development.
Impaired Flower Bud Development:
- Flower bud differentiation: Almond trees initiate flower bud development during the fall and winter. High day/nighttime VPD can disrupt this process, leading to:
- Reduced flower bud formation: Fewer flower buds develop, potentially resulting in lower fruit set and yield.
- Weak flower buds: Even if buds form, they may be weaker and more susceptible to damage from frost or other environmental stresses.
Reduced Fruit Set and Yield:
- Pollination: While almond pollination primarily occurs during the day, high nighttime VPD can indirectly affect pollination viability and consequently success. Stressed trees with limited carbohydrate reserves might produce fewer or less viable pollen grains, reducing pollination potential.
- Fruit Development: If flower bud formation and development are compromised by high nighttime VPD, it ultimately translates to a lower number of fruits set and potentially smaller fruit size, leading to lower overall yield.
Additional Considerations:
- Water Stress: High nighttime VPD can exacerbate water stress even if irrigation is adequate. The increased “pull” on water molecules at night can deplete plant water reserves, further impacting daytime performance.
- Increased Susceptibility to Disease: Water-stressed trees are more vulnerable to fungal diseases, and high nighttime VPD can create a favourable environment for fungal spore germination and infection.
Strategies to Mitigate High Day/Nighttime VPD:
While controlling day/nighttime VPD directly is challenging, some strategies can help minimize its negative impacts:
- Irrigation Management: Ensuring adequate soil moisture before nightfall allows trees to access readily available water at night, reducing the stress caused by high VPD. If you’re expecting high night VPD values for your almond orchard, prioritize irrigation in the late afternoon or early evening. This will help them better cope with the upcoming evaporative stress. Misting or spraying water above the almond canopy can be a valuable tool for reducing high nighttime VPD. This technique works by increasing humidity and lowering temperature, creating a more favourable environment for the trees.
- Canopy Management: Techniques like promoting good air circulation within the orchard can help reduce nighttime humidity buildup, leading to slightly lower VPD around the trees. However, nighttime canopy manipulation strategies are limited compared to daytime options.
- Selection of Drought-Tolerant Cultivars: Choosing almond varieties known for better water use efficiency and drought tolerance can be beneficial in areas with frequent high nighttime VPD.
Monitoring and Early Intervention:
Monitoring nighttime VPD levels along with other environmental factors like temperature and humidity helps growers identify potential risks and implement strategies to mitigate their impact on almond trees. By taking proactive measures, growers can improve tree health, flower bud development, fruit set, and ultimately, maximize their almond yields.
Yes, High Nighttime VPD Can Reduce Plant Tissue Rehydration
Here’s how high nighttime VPD hinders plant tissue rehydration in almonds and the concept of threshold values:
Mechanisms:
- Increased Transpiration: Even with stomata partially closed at night, high VPD can still create a strong pull on water molecules within the plant. This can lead to continued, albeit reduced, transpiration, causing some water loss throughout the night.
- Reduced Water Uptake: While water uptake from the soil can occur at night, high VPD can limit the rate of water movement within the plant. This is because the “driving force” for water movement (the pressure gradient between soil and plant) is partially reduced due to the drier conditions (higher VPD) within the plant.
Consequences for Rehydration:
- Limited Nighttime Recovery: Due to the factors mentioned above, high VPD can prevent the plant from fully rehydrating its tissues at night, following water loss during the day.
- Accumulated Water Stress: Over time, this incomplete nocturnal rehydration can lead to accumulated water stress in the plant, impacting its overall health and performance.
Threshold Values:
Unfortunately, there isn’t a single, universally accepted threshold VPD value for nighttime rehydration in almond trees. It can vary depending on several factors:
- Almond Cultivar: Different almond cultivars may have varying tolerances for nighttime VPD based on their inherent water use efficiency and drought resistance.
- Plant Age and Health: Younger or weaker trees might be more susceptible to rehydration limitations at lower VPD levels compared to mature, healthy trees.
- Phenological stage: The sensitivity of fruit trees, including almonds, to VPD varies depending on their growth stage. Flowering, fruit set, and fruit development are particularly vulnerable periods, where high VPD can have the most detrimental effects on crop yield.
- Yield: The amount of fruit on a tree can influence its susceptibility to VPD. Trees with heavier fruit loads may be more vulnerable to the negative effects of high VPD.
- Environmental Conditions: Other factors like nighttime temperature and humidity can influence the impact of VPD on rehydration. For example, a high VPD combined with high nighttime temperatures would create a more significant rehydration challenge than high VPD with cool nighttime temperatures.
As a general guideline, night VPD values exceeding 0.6 Kpa can potentially cause plant stress, although the specific impact may vary depending on the factors discussed earlier.
The use of foggers or sprinklers at night to address high nighttime VPD in almond trees:
Potential Benefits:
- Increased Humidity: Foggers and sprinklers directly add moisture to the air, raising the relative humidity (RH) around the trees. This reduces the VPD, lessening the “pull” on water molecules within the plant and minimizing water loss through transpiration at night.
- Improved Rehydration: By creating a more humid microclimate, foggers or sprinklers can potentially enhance nighttime rehydration of plant tissues, counteracting the drying effects of high VPD.
Limitations and Considerations:
- Disease Risk: High humidity at night can create favorable conditions for fungal spore germination and disease development. This is a major concern for almond trees, which are susceptible to fungal diseases like Botrytis blight.
- Water Management: Foggers and sprinklers can use significant amounts of water. Careful monitoring and application techniques are crucial to avoid overwatering and potential root rot issues.
- Leaf Wetness Duration: While some nighttime leaf wetness can be beneficial, prolonged periods can also promote disease growth. Balancing the rehydration benefit with minimizing wetness duration is important.
- Cost and Infrastructure: Setting up and operating fogger or sprinkler systems requires additional investment and ongoing maintenance.
Foggers vs. Sprinklers:
- Foggers: Generally considered a more targeted approach, creating a fine mist that increases humidity without excessive water application. However, foggers can be more expensive to install and operate compared to sprinklers.
- Sprinklers: Offer a simpler and potentially less expensive option for raising humidity. However, sprinklers can deliver more water than necessary and lead to longer leaf wetness periods, potentially increasing disease risk.
Overall:
Using foggers or sprinklers during high nighttime VPD can be a strategy to consider, but it requires careful evaluation of the potential benefits and drawbacks. Disease risk management and efficient water use are key concerns.
The optimal approach likely involves a combination of strategies depending on the specific needs of the orchard, environmental conditions, and water availability.
Research and Monitoring:
While specific threshold values are elusive, ongoing research is helping to refine our understanding of how VPD interacts with other environmental factors and plant characteristics to impact rehydration. Growers can benefit from monitoring nighttime VPD levels along with other environmental parameters to assess potential rehydration risks and implement strategies like irrigation management to mitigate them.
In conclusion, high nighttime VPD can hinder plant tissue rehydration in almond trees, but the specific threshold values for concern depend on various interacting factors. Ongoing research and monitoring practices are crucial for optimizing almond tree health and performance in the face of fluctuating VPD conditions.
Wind’s Impact:
- Increased water loss: Strong winds significantly raise transpiration rates, leading to water stress for the trees. This can exacerbate the negative effects of extreme temperatures, both low winter chills and summer heat.
- Pollination disruption: Similar to rain and fog, strong winds hinder bee activity, negatively impacting pollination rates.
- Physical damage: High winds can cause significant physical damage to the orchard, including:
- Flower and fruit drop
- Branch breakage
- In extreme cases, entire trees can be toppled (Arquero, 2013)
Wind Management Strategies:
- Windbreaks: Planting windbreaks, such as rows of trees strategically positioned around the orchard perimeter, can help shield the grove from strong winds.
- Planting design and pruning: Careful orchard design that promotes good air circulation and appropriate pruning techniques that reduce crown density can help minimize wind resistance and potential damage.
Soil Texture and Drainage:
- Heavy clay soils: These can hinder root growth due to poor aeration and drainage. While they retain water and nutrients well, this can also lead to waterlogging, which can suffocate roots. Almonds are particularly sensitive to this. To avoid these issues, consider:
- Using rootstocks resistant to waterlogging or with shallow root systems.
- Installing drainage systems before planting.
- Planting on raised beds to improve drainage.
- Silty soils: Similar to clay soils, they have poor structure, leading to crusting and erosion.
- Sandy soils: While these soils are well-aerated and promote root growth, they drain quickly and have low water and nutrient retention capacity.
Effective Soil Depth:
The depth of usable soil for the roots is crucial, as it impacts water and nutrient availability. Factors limiting effective depth include:
- Hardened horizons: These can be caused by calcium carbonate accumulation (petrocalcic), clay accumulation (argillic), or the presence of bedrock.
- Shallow water tables: High water tables can also restrict root development.
Limited depth can hinder tree growth and production, especially in areas without irrigation.
Improving Effective Depth:
- Deep subsoiling: Breaking up compacted layers or petrocalcic horizons before planting can significantly increase the usable soil depth for roots.
Overall:
While almond trees can tolerate some soil limitations, deeper, well-drained soils with good aeration will promote better root development, leading to healthier trees and higher yields.
Chemical Properties for Optimal Growth:
Several key chemical properties influence almond tree health and productivity:
- Organic Matter: Ideally, soil organic matter content should be around 2%. However, many almond-growing areas typically have levels between 1-1.2%. Organic fertilization practices can help improve this crucial soil component.
- Carbonates: Moderate levels of carbonates (up to 20%) can benefit soil structure and microbial activity. However, excessive concentrations (>20%) can limit the effectiveness of fertilizer application.
- Active Limestone: Fine, highly reactive carbonate particles exceeding 9% can hinder normal almond tree development.
- pH: Soil pH significantly impacts nutrient availability, microbial activity, organic matter decomposition, and cation adsorption. Almond trees can tolerate a wider pH range (5.5 to 8.4) compared to some crops.
- Salinity: Measured by Electrical Conductivity (EC), salinity reflects the concentration of major ions (cations like sodium, calcium, and anions like chlorides) in the soil. While almonds are relatively salt-tolerant (up to 4 dS/m EC), yields begin to decline above 1.6 dS/m EC.
- Nutrient Levels: A C/N ratio of 10-12 and a total nitrogen content (Kjeldahl method) of 0.11-0.2% are considered optimal for almond trees.